The evolution of animal forms is usually told as a story. Here’s one: a long time ago, our fishy, jaw-less ancestors had gills that were stiffened by bony arches, and over time, the front two pairs of these evolved into the lower and upper jaws of most animals, including ourselves. Of course, this story is told backwards because figuring it out really started from today’s creatures and went backwards to older and older ancestors. For vertebrates like us, there are myriad stories that trace the origin and evolution of just about every part of our bodies back hundreds of millions of years to some ancestral structure in some fish-like creature. The evidence for these stories comes in part from the fossil record, in part from the reasoning about comparative vertebrate anatomy and in part from embryology (the development from fertilized egg to an independently-living animal). During embryonic development of animals, structures generally arise much as they did in evolution, thus development illuminates evolution, although no one takes this claim quite as literally as they used to. Accepted evolutionary stories “make sense” (one of science’s most dangerous claims), that is, they are reasonable, coherent and credible.
When I studied comparative vertebrate anatomy in college, one of the most repeated stories traced the evolution of the long bones (humerus, radius, ulna, etc.) of tetrapod (four-legged animal) limbs back to the little bones in the fin bases of the mostly extinct fleshy-finned fishes (in contrast to most modern fish, which are ray-finned), of which lungfish and coelocanths (see below) still survive . This story is supported and illustrated with a pile of fossil and comparative morphological evidence, and was one of the glories of the field, uncontested for a couple of centuries.
Not so for all the small bones in the five-digit hands/feet of these same tetrapods—- yes, they could be traced to a five-digit early tetrapod ancestor and therefore go by the same names, but they cannot easily be traced to that much earlier fleshy fin that gave rise to the long bones. The story has jumped a big puddle, and suddenly we’re on the other side and we don’t know how we got there. You look for a bridge, and you can’t spot one, but maybe if you look long enough, one will turn up.
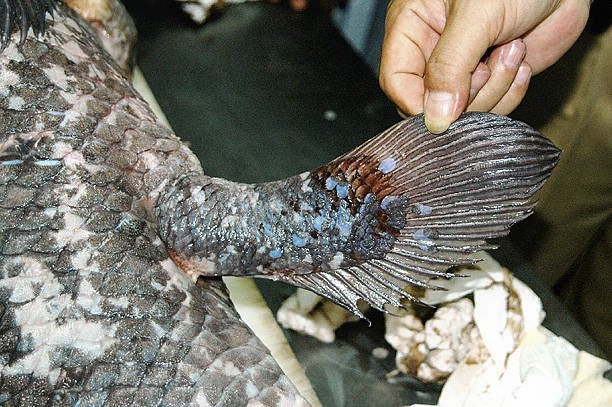
My Found Object for this essay is part of this missing bridge story. I came upon this object at the inner margin of a salt marsh on the Florida coast--- a strew of bones that once belonged to a bobcat---- skull, ribs, claws, pelvis, vertebrae, and limbs. The absence of the epiphyses (growth plates) from the long bones, as well as the separation of the pelvis into pieces told me that this bobcat had died young.
To me, very small, delicate bones are aesthetic objects, so I picked out the bones of the cat’s feet and left the rest to decay and slow burial. The sculpted bones made quite a neat, pretty little pile, like the work of an artist.
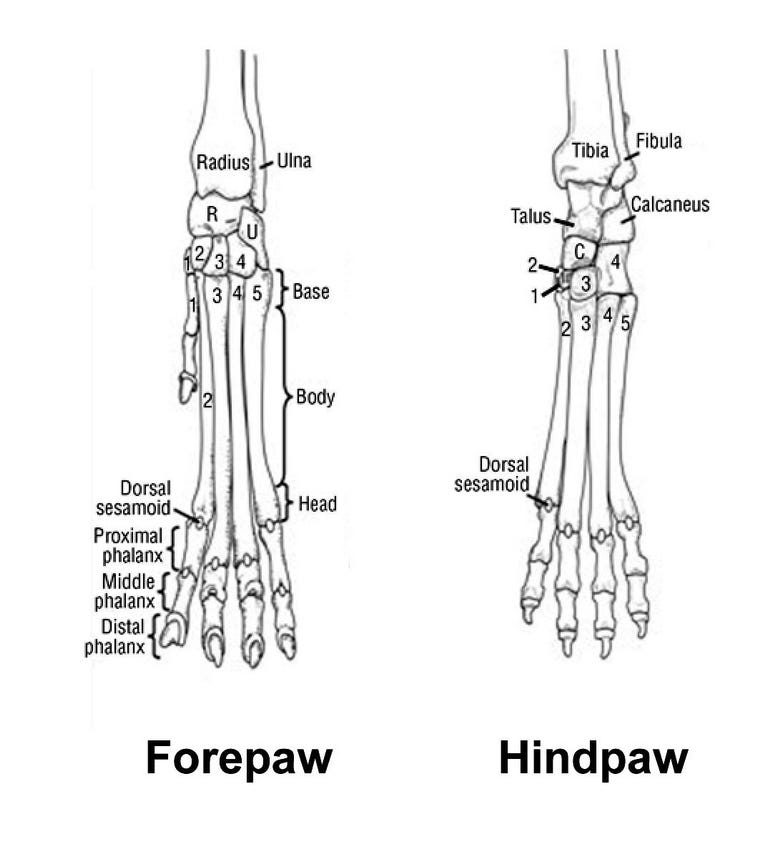
As a college student, I loved how the story of limb evolution came together, especially when I learned that a few years previously, a “living fossil” fish, a coelocanth, belonging to a group long thought to be extinct, had been caught off Madagascar, and it had the fleshy fin bases that were said to be the great-great granddads of tetrapod limbs. So, what luck that my first job was at Rhodes University in South Africa, and that the two people responsible for bringing the coelocanth to science both had affiliations with Rhodes! It was a memorable day when my friend Cliff asked if I would like to see the actual, original coelocanth. He lifted the stainless-steel lid off a tank the size of a large bathtub, and there, swimming eternally in its formalin sea, was a strange fish almost as large as me, its fleshy fin bases clearly visible.
The story of the discovery of the coelocanth began in 1938, when Miss Marjorie Courtney-Latimer, a museum curator in South Africa, was notified by a local fisherman of a large, strange fish in his catch . Being no ichthyologist, she made a sketch and sent it to J.L.B. Smith, an ichthyology professor at Rhodes University. Unfortunately, Smith was away, and failing to receive an answer, she hauled the fish to her museum in a taxi, and tried to identify it from books, but to no avail. Since the fish was starting to rot, and the morgue wouldn’t help her, she had a taxidermist stuff it, thereby losing all the internal structures. When Smith returned, he immediately recognized it for what it was, and named it Latimeria chalumne in honor of Latimer. However, it was 1952 before a second specimen was caught, and this time, its fate was not the taxidermist, but the formalin bath in which it was still swimming when I saw it.
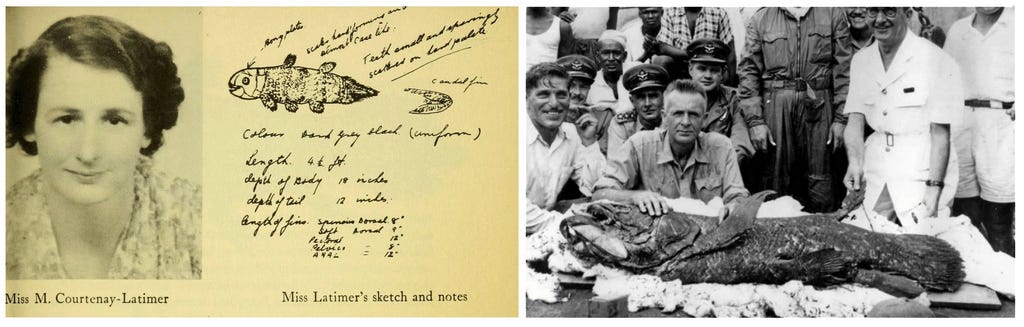
But now, back to the “missing bridge” in the story of how a fleshy-fin of an ancient fish ancestor evolved not only into tetrapod limbs and their bones, but also somehow gave rise to the hand. As I said above, the first part of the story was cinched by a long line of fossil intermediates as well as embryonic development, but no part of these ancestral fins bore a resemblance to hands, being composed as they were of many thin, ray-like bonelets arranged like the branches of a fir tree (middle image below). How did this mess evolve into the neat tetrapod hand/foot with its exact number of bones and five digits?
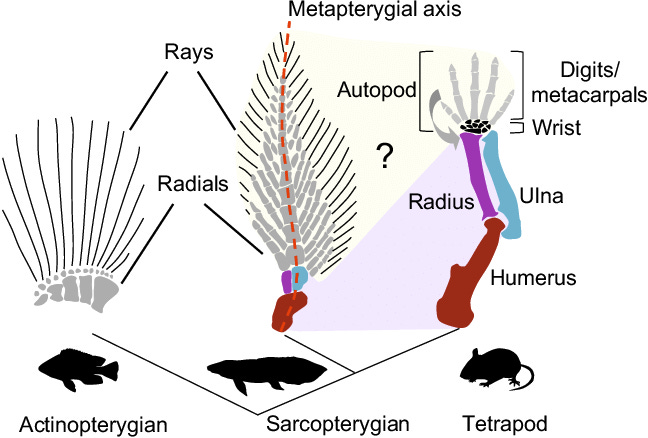
Fossils and comparative anatomy failed to provide an answer, but as already noted, if you wait long enough, a bridge materializes. The bridge was provided by modern genetic evolutionary development, aka “evo-devo.” Traditional embryology originated two centuries ago with the first descriptions of the stages in the development of an animal, from fertilization to adulthood. Many a biology major, including myself, has studied chick and frog embryos of increasing ages to understand this unfolding of form. I suspect I was one of the few who really enjoyed it.
With the development of modern molecular genetics, it became possible to identify the genes that were activated at each stage of development. The manner in which genes establish the basic body plan turned out to be surprisingly simple. Across all animals, the same set of genes, aka the “evo-devo gene toolkit” or hox genes orchestrates early development, first establishing the anterior-posterior axis, then activating different toolkit genes depending on the location along this axis. This initiates a cascade of gene activity and their products that cause the structures appropriate to each body region to grow and take form. The genes do this by producing transcription factors, that is, specific proteins that switch on specific genes and start the cascade of gene activity that determines cellular fates. The diagram below shows how this works in the fruit fly, Drosophila, the best studied creature in this regard. You may well ask, why are you showing me a fruit fly when were were talking about tetrapods and their fleshy-finned ancestors? No matter, the genes, and the way they work are the same.
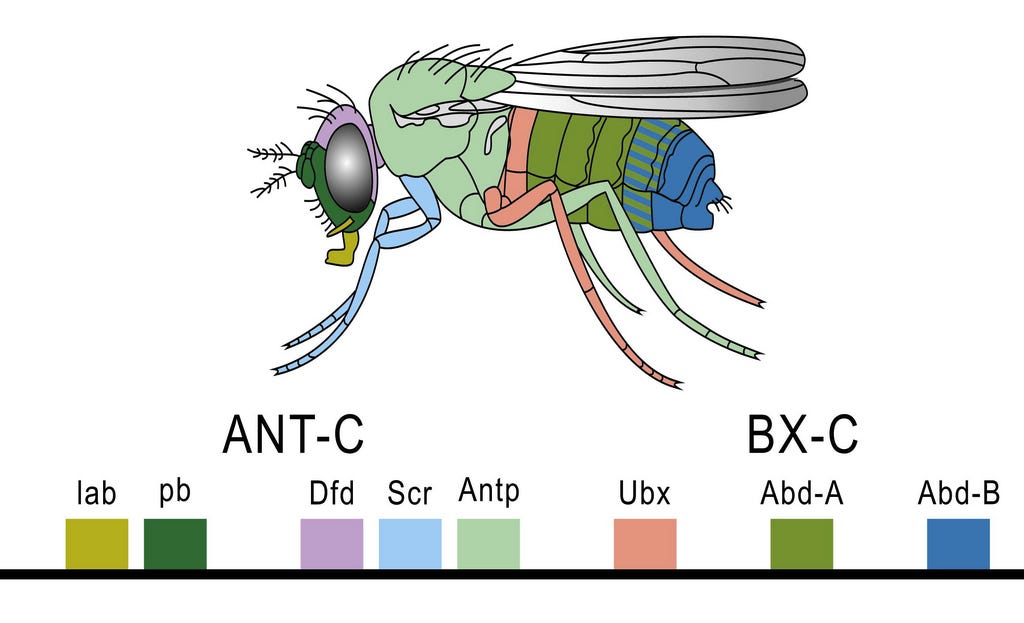
By producing transcription factors in different amounts, a zone may become more or less of whatever its fate is to be. Thus, for an extreme example, the toolkit genes that cause limbs to grow in the right locations and sizes in most tetrapods are inactive in snakes, that is, they produce little or no transcription factor, and thus limb growth and differentiation do not take place. Snakes evolved to be limbless through the modification of particular toolkit genes, and this profound principle applies to all differences in animals’ body plans. As the cascade continues, ever more local genes are activated as the embryo becomes more and more complex and its structures begin to approach their final form.
Could the pattern of gene activation be the bridge that tells us how the fins of fleshy-finned fishes evolved into the tetrapod hand/foot? Yes, it can, if we compare the patterns of gene activation during fin/limb formation in a suitable fleshy-finned fish with that of a tetrapod, such as a mouse. After all, they are the same genes activated to differing degrees.
Sadly, the beloved coelocanth that connected the cat’s toe bones with my time at Rhodes University turned out to be, not an ancestor, but an offshoot from the main evolutionary line leading to the tetrapods, and was, so to speak, a red herring. Instead, another fleshy-finned fish, the Australian lungfish shares a more recent common ancestor with tetrapods, and is a whole lot easier to work with. Australian lungfish live in slow moving streams in, you guessed it, Australia, growing to a maximum size of 50 kg and 1.5m in length, and living for more than a century. If you want to buy one legally, it will cost you over $1000 for a small one, and it will come with a microchip that identifies it as having been legally purchased under the Australian conservation laws. Of course, if you want fertilized eggs, you need a boy and girl fish as well, but be warned that they don’t mature until they are 15 years old, much like human children. Or you can just buy fertilized eggs from a fish farm.
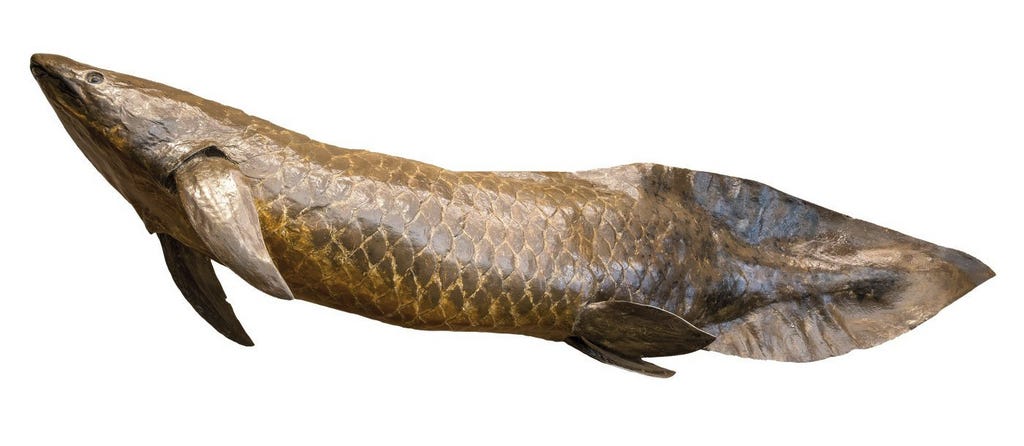
With developing eggs now in hand, it is time to look at how the hox and other toolkit genes spring into action in the embryonic pectoral fin and compare these patterns with those acting in the mouse front leg/hand. After all, the same conserved hox genes orchestrate the building of both. So, how hard could it be to mark all the cells in which each hox gene is busily transcribing? Actually, very hard, and you need a lot of expensive equipment and supplies, not to mention some mighty advanced knowledge and skill.
Still, it can and has been done. Simplifying a great deal, because the DNA sequences of all the hox genes are known, you can make primers (shorter pieces of DNA) whose sequence represents part of each hox gene, and will therefore bind to messenger RNA made by these hox genes. To these primers, you attach a strong antigen, a favorite being derived from the flower foxglove, or you can tag the primer with fluorescent or colored dyes. OK, you are now ready to start, so after you make the tissue (in our case, the embryonic pectoral fin or mouse limb) more permeable, you bathe it with the tagged primer so that wherever the target hox gene has actively produced messenger RNA, the tagged primer will hybridize (bind) with it. If you used an antigenic tag, you bathe the prep with fluorescent-tagged antibody. You then wash out all primers that have not been hybridized. Finally, you let the magic happen--- wherever the primer is bound, its fluorescence or color marks the cells in which the hox gene corresponding to your primer has been active, ready to be photographed and studied. If you want to get fancy, you can use double labeling with a different color for each hox gene. Simple, right?
What you get is a set of images like the example of a late-stage lungfish pectoral fin below that shows where several toolkit genes are transcribing their factors, initiating the cascade that will cause the fin to develop into its final shape. Detection for col2a is in red, the central spine gene in azure, and the hoxa13 in green. Other preps for other stages and genes paint a more complete picture of changing patterns of gene activity that give the fin its anterior-posterior, basal-distal, and central spine shape.
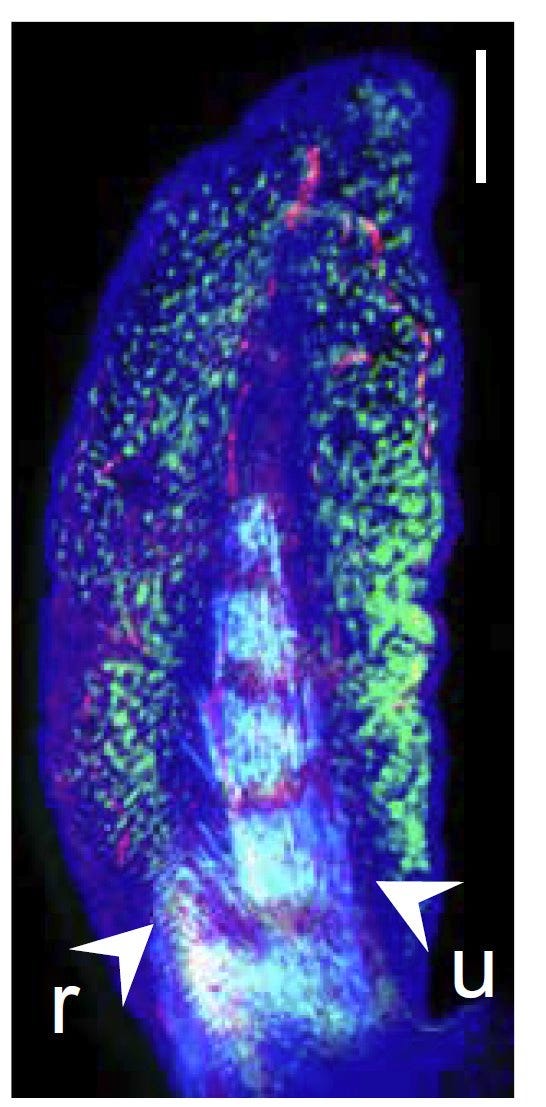
How does this compare with the same genes that orchestrate the development of the mouse forelimb and hand? In the image below, the authors interpret how differences in the hox genes’ activity lead both to the lungfish fin and the mouse wrist/ hand. The whole hand of the mouse is homologous (shares a common ancestry) with only the posterior region of the lungfish fin, a consequence of the hyperactivity in the mouse of the posterior hox genes (blue) and reduced activity of the anterior genes (green). The fingers are homologs of five of the posterior fin-spines. In effect, the central axis of the spine in the lungfish fin has been shortened and bent forward to form the bones of the wrist (the active hox genes in the image are identified in the box and by colors). Presumably, the hands and limbs thus created were associated with the transition to land as fleshy-finned ancestors became primitive amphibian tetrapods.
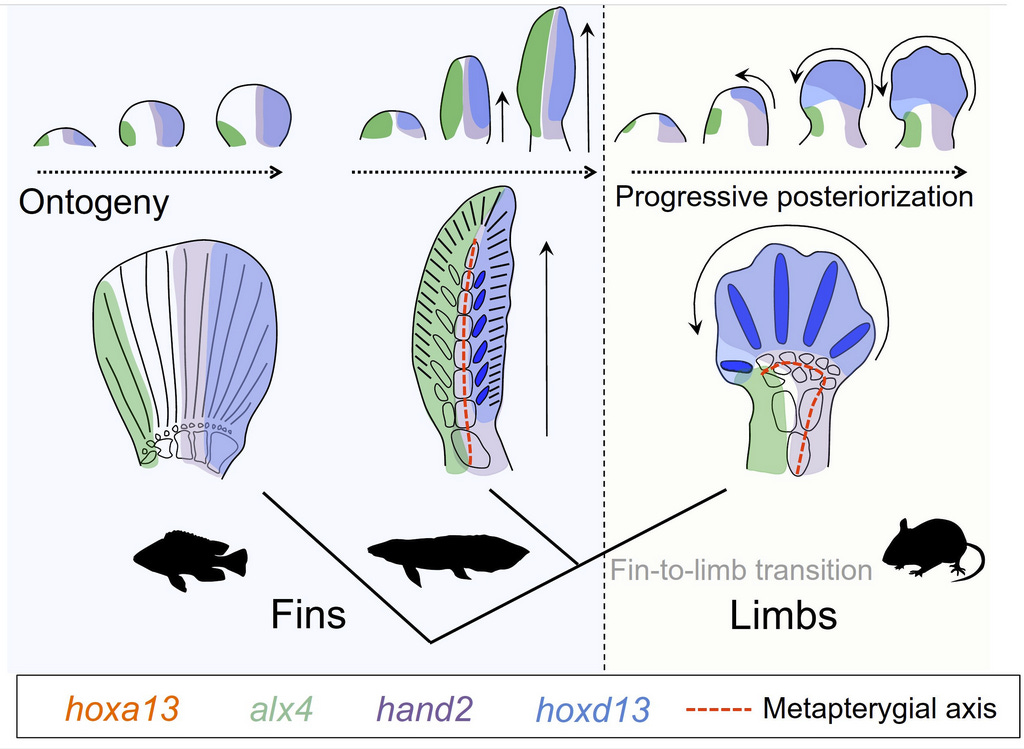
So, here we are then, a long time later and distance farther. A little pile of cat foot bones has taken us on a roundabout cruise through comparative vertebrate anatomy with a detour to the coelocanth at Rhodes University, and then to Australia’s lungfish, followed by the thicket of evo-devo and how genes direct the formation of fins and hands. In a sense, this is the same journey that the science of vertebrate anatomy/development/evolution has made. In college, it was fascinating and fun to dissect, inspect and detect animal structures, and this simple and direct process answered a lot of important questions, both for me and for science. But the answers were limited, offered in large chunks with little detail at lower levels. The astounding progress of molecular genetics applied to animal development began to reveal how genes directed both development and morphological evolution. All this happened in the 60-plus years since I dissected that dogfish shark on a slate-topped lab bench in the old-fashioned Shanklin Laboratory with its ten-foot-high windows. Now, a lifetime later, I struggle to understand, in vastly greater detail, what has been learned since then about how animals evolved and developed. It is said (yes, it is!) that the amount of scientific knowledge has increased ten-fold in the lifetime of every scientist that has lived in the last 400 years. I find that claim easy to believe.
Thanks for another fascinating essay. You deserve a big hand!
This was very helpful for this reader, who knew only the broadest outlines of the picture. Has anyone studied Hox moderated processes in non tetrapods, like the mollusca?