In a previous post, I described a contraption that helped me identify the cues that prevented crowded tenebrionid beetle larvae (Zophobas rugipes) from pupating. A series of experiments showed that the cues were not chemical or diet-related, but were the tactile stimulation that resulted from larvae crawling over larvae. This stimulation translated into the physiological/hormonal inhibition of metamorphosis.
Having answered how crowding inhibited metamorphosis, raised the question of why, a much more difficult question for science. The first hint of why came from noticing that every once in a while, a larva would find a quiet corner and go into pupation mode (which is immobile and nearly defenseless), but within a day or two, other larvae would find and eat it. Moreover, isolating larvae long enough to begin pupation, and then returning them to the madding, munching crowd inevitably turned them into lunch for active larvae. Given such a fate, natural selection should quickly eliminate larvae that pupated in crowds rather than in private. But was this lab situation also true in nature? After all, the beetles didn’t evolve in my lab.
My brother was living in Honduras at the time. Two entomologists working at the Pan American Agricultural School in El Zamorano, Honduras told him that bat guano and my tenebrionid beetles regularly drizzled from the attic onto their desks. So here was my chance to see how it all worked in nature (an attic is a pretty good facsimile of a cave).
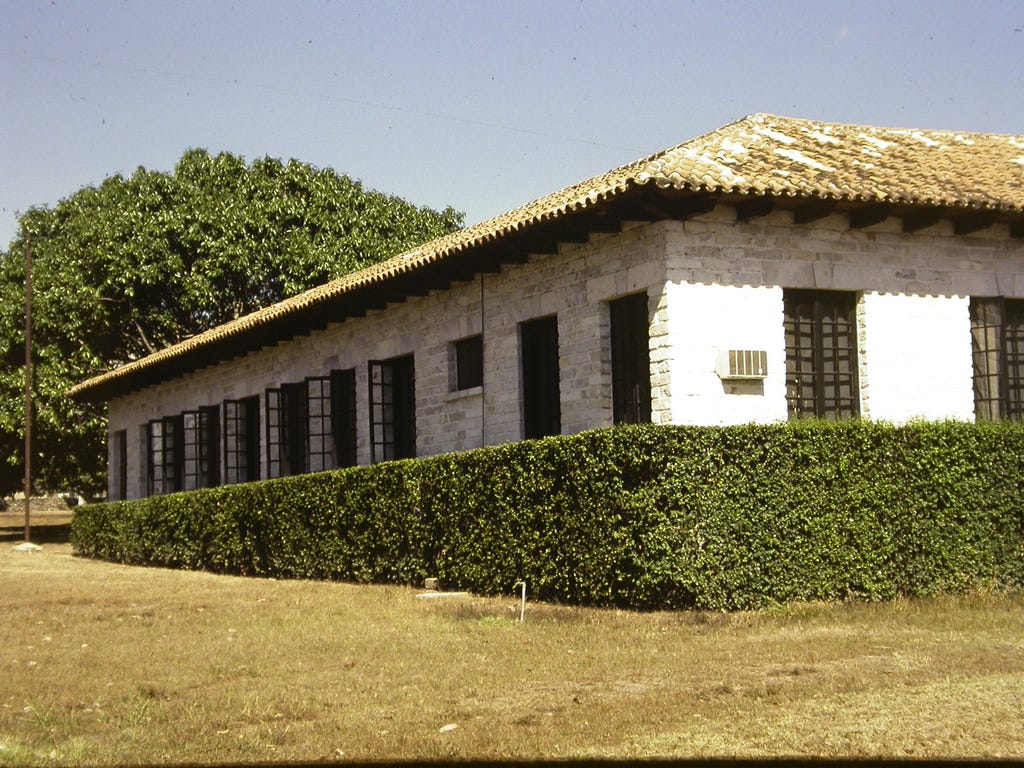
So, for several days, I wallowed in bat guano, dust mask donned, and rabies shots up to date, observing and testing what the larvae did in this pile of guano. To begin with, they weren’t on a strict guano diet, for any bat that fell off its perch and died was quickly skeletonized by the larvae. So, the guano was, by today’s opinions, a “healthy” diet, having been produced by fruit bats and several other species with a range of diets, including insectivorous as well as vampire bats. Vegan larvae were unlikely.
The main mass of guano was a wiggling crowd of active larvae, but around the thinner perimeter and in nooks and crannies away from the pile, there were many in the process of pupation. If I moved these to the main pile, they were invariably and quickly eaten. Adult beetles that had completed metamorphosis away from the pile returned to the pile to reproduce. What happened in nature had thus confirmed my lab observations. Larvae could survive the vulnerable pupation stage only by shunning the crowd and finding a peaceful nook without their cannibalistic brethren (sistren?).
Because a well-adapted life history depended on body weight and age at first reproduction, the larvae were making choices of crucial importance to their lives: when to stop feeding and tolerating crowding, when to disperse away from food and the larval crowd, how to tell when they had found a quiet, safe spot, and whether to occupy that spot passively or defend it actively. These life and death decisions were made by larvae that were largely a mobile digestive system under the command of a tiny brain and a few hormones. No college degree required.
I needed to measure the strength of their enthusiasm for dispersal and isolation, and to do so, I devised another gizmo, the so-called Dispersarium. Made of Plexiglas, it consisted of a large, round, central arena, surrounded by 26 small chambers that each connected to the arena by a short tunnel. The idea was simple--- if the pupation chambers were in short supply, the larvae should compete for them, and if there were plenty to go around, peace should reign, and every larva should have a quiet place to pupate. Unlike the Stimulatorium, the Dispersarium had no moving parts.
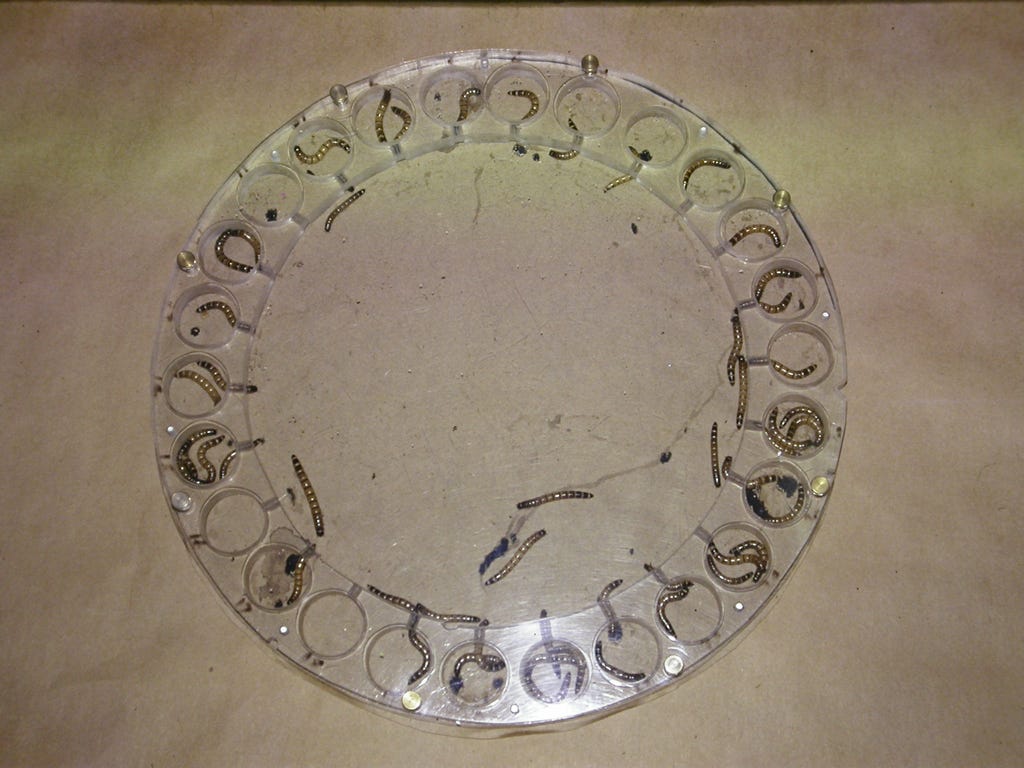
But how could the Dispersarium tell me whether the larvae were trying to disperse, to aggregate, or were just entering chambers randomly without interacting, in other words, that the distribution of larvae among chambers was or was not generated by chance? At this point, a popular mathematical game came to the rescue, The Birthday Problem. Say, you have invited a number of people to your party, and during happy hour you ask how many of your guests have a birthday on the same day. The Dispersarium is like your party game, because just as the year has only 365 days, the Dispersarium has only 26 chambers. We are simply asking, what is the chance that two birthdays fall on the same day, or that two larvae (for example) are in the same chamber. Of course, we can also calculate the chance that zero, one, two, three, and so on birthdays or larvae occur together (most people are surprised by the frequency of shared birthdays).
If the occupancy of Dispersarium chambers is purely chance, the “party” in the Dispersarium will follow the Birthday Problem. However, if there is interaction between the larvae, such as attraction or repulsion, the distribution of larvae will deviate from the random, and the nature of this deviation will tell us the nature of larval interaction.
Whereas birthdays are randomly distributed across the year, my lab and attic observations suggested that both larval age and crowding should affect dispersion among chambers. To test crowding, let’s add 13, 26, and 52 larvae to the Dispersarium, and to test age (actually weight, but that is tightly related to age), let’s make these larvae 250, 500, and 1000 mg in weight (i.e. small, medium, large). Our measure of dispersion is the difference between the observed number of chambers with zero, one, and two or more larvae and the number expected by chance (the birthday problem). We will express this difference as a “dispersal index”--- when this index is greater than one, the larvae are aggregated with more empty chambers and more chambers with two or more; when it is less than one, there are more single-occupant chambers, fewer empty chambers, and fewer chambers with two or more (i.e. they are more evenly distributed, or "overdispersed.")
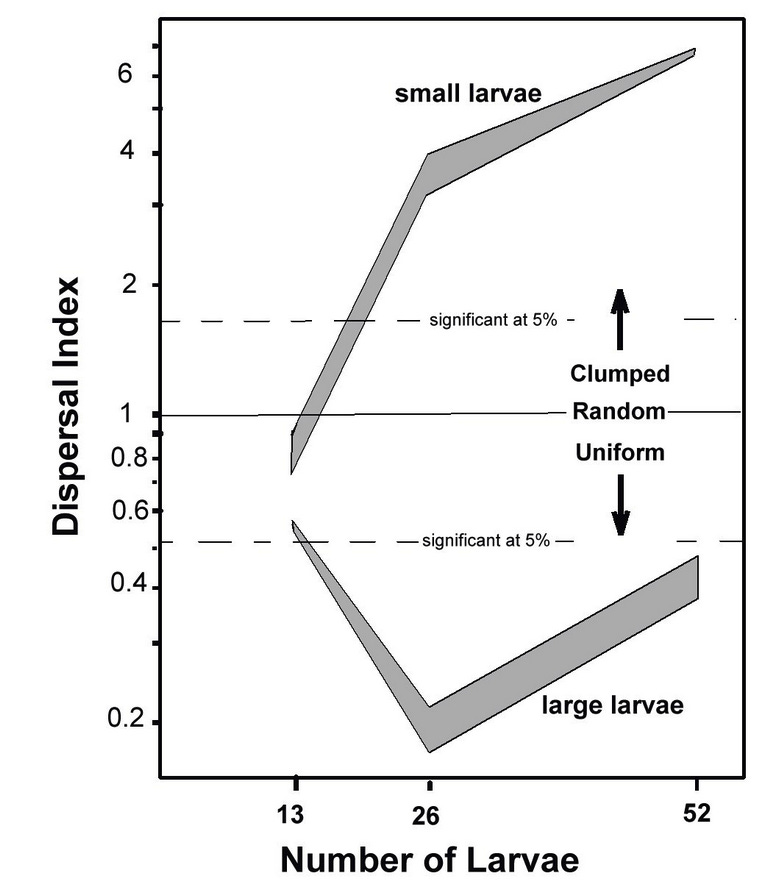
No matter how many larvae we dump in the central arena, they always skitter quickly to the perimeter and enter the chambers. They clearly prefer chambers over the wide-open arena. There is some early rearrangement, but this stabilizes after a few days. In the graph above, you can see that when we add 13 larvae of any size, they distribute themselves randomly because there are more chambers than larvae. With 26 or 52 larvae, the dispersion differs greatly by size. Small larvae that are not yet capable of pupation aggregate in a few chambers, so that there is a great deficit in chambers with one larva and a great excess in chambers with zero or two or more larvae.
Large larvae, those of a size capable of pupation, quickly establish an excess of chambers with a single larva and a deficit of chambers with zero or two or more. They do this by placing themselves in or near the entry tunnel, and attacking and driving off any other larva trying to get in. There is a lot of pushing, biting, and thrashing. After three or four quiet days of sole occupancy, they curl up in the pharate pupa position and begin metamorphosis. With 26 larvae, almost all end up alone in a chamber. Apparently, they did not learn that "sharing is caring" in elementary school.
When there are 52 larvae, nearly half are not able to occupy a chamber, and spend their time in the arena, often remaining active and not pupating. After three to four days when the larvae in chambers have become defenseless pharate pupae, an active larva from the arena may occasionally enter a chamber, cannibalize the occupant, and then itself enter the pharate stage.
The response by medium larvae is mixed, probably because some are capable of pupation, and the remainder are still seeking aggregation, leading to high variation in dispersal.
To summarize these patterns as a narrative description, small larvae either don’t care about crowding, or seek it out because it promises to be associated with a pile of food. Once they reach a size capable of pupation, they become increasingly averse to the tactile stimuli of crowding, and disperse away from the feeding crowd and its cannibals to seek a quiet, preferably protected spot where they can safely pupate. The absence of the inhibitory tactile stimulation then allows the hormonal, physiological, and developmental changes of metamorphosis to proceed.
So, by means of two contraptions, we first established why crowding inhibits metamorphosis, and then established how and when the larvae deal with this inhibition in order to transform into adults capable of reproduction. The timing of these larval choices is the raw material through which natural selection adjusts how long larvae spend as larvae, how large they grow before pupating and reproducing, what their absolute age is when they first reproduce, and how all of these are affected by how old their mother was when she laid the egg that produced them.
The trouble with delving deeply into these questions is that it leads to very large, complex experiments, bristling with complex statistics, and requiring an exhausting amount of work and time. I succumbed to the temptation to complete such a multi-generation experiment requiring a precise routine and detailed organization over several years. After the simple path from the first observation of the effects of crowding, to the Stimulatorium and the Dispersarium, I now found myself in the experimental equivalent of a forest.
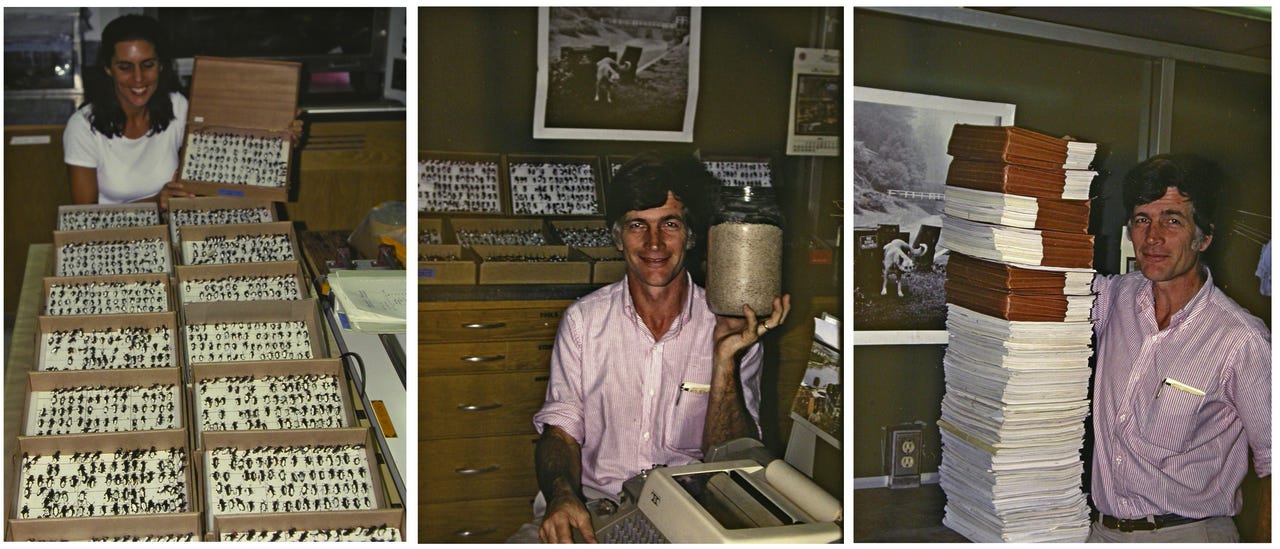
I pride myself in having completed that project, but the simple joy of the early experiments was replaced by regimented experimental schedules, and the processing and analysis of data. It is probably this shift from joyous exploration to bureaucratic competence that explains why it took me 12 years to publish the results of this “forest” experiment. I learned a great deal about data analysis and statistics, all of which have stayed with me since, but I also learned that in choosing a research project, fun, directness, and simplicity can be attractive criteria. Sadly, in spite of having "learned” this lesson, this was not the last colossal, complex project I undertook. Perhaps I am a slow learner, or a poor judge of what the future will require, but were I not, my scientific accomplishments would be a good deal smaller.
An Addendum: After writing this essay, I became curious if there had been follow-up to my research, and did an online search for Zophobas. I was astounded by what I found. Since the year 2000, there have been between 100 and 250 publications per year on this insect, on subjects ranging from endocrinology of metamorphosis, to defense against cannibalism, to mass rearing as feed for fish, chickens, and humans. The larvae are sold as “superworms”, with several dozen businesses that will sell them to you a few at a time or by the bucket full, some with free shipping. The irony of this is that about 30 years ago, I was asked by the Florida Department of Agriculture to comment on a permit application for rearing them in the state. Did this insect have potential to become a pest, they asked. I said that in view of its biology, it had a potential as a stored products pest. I guess they weren’t too impressed by my judgement, and now, “superworms” are reared all around the world on a range of organic stuff. Most recently, these larvae were reported not only to eat Styrofoam, but to digest it as well, aided by gut bacteria. I knew this critter was a winner right from the outset when I collected them from that pile of bat guano.
Though Zophobas rugipes is a very worthy subject of study with an enormous amount of things to be learned (effects of heat and humidity in dispersal, selection issues in generating thicker "skins" for defense against cannibalism, motility issues in dispersal (i.e how far are they willing to travel) etc.etc.etc.) still, I'm glad that Professor Tschinkel's eventually concentrated on the ants. I see the fascinating aspects of beetles- all life forms are fascinating- but the ants are my own faves. 🐜🐜🐜 On the side, were the dispersariums configured with varying depth/size chambers? Getting more complex, perhaps a central arena with 3 passages to 3 sub arenas, each sub arena with 3 passages to pupation chambers? Then introduce variables of light, heat, etc. Really interesting stuff! Are the critters willing to travel uphill? Downhill? To what angles?
What a critter! It may help to save the world.