Eating blue crabs is popular wherever you can buy them. Lazy people buy just the meat that has been picked from the cooked crab and packaged. But at parties, hosts often serve only the blue crab claws, aka chelipeds. Sometimes you are expected to crack the thick shell yourself to expose the edible muscle within, but sometimes the host has removed part of the shell as in the image below. The pinkish stuff is the muscle that you are going to eat.
Crabs use their claws for a range of manipulations, from crushing, pinching to delicately picking stuff up. To do this, the top claw (the dactyl) can be pivoted in relation to the fixed lower claw using the muscles you see. The pictured claw is the right claw, aka the “crusher” for breaking open mollusk shells and other hard stuff (note the large crushing teeth). The left claw (not pictured) is the less strong “cutter” and is used for picking out stuff from the crushed item. In the great majority of crabs, crushers are the right claw and have more powerful muscles than the cutters on their left.
If you are like most crab-eaters, you will pay no attention to what is left once you have scraped this muscle into your mouth, and you will discard the leftovers as trash. This is too bad, because the whole claw apparatus is a marvel of engineering, a machine of levers that appropriately proportions force and movement. Because the fact is so commonplace, it does not often rise to the level of consciousness that this little machine is faithfully replicated in every blue crab alive and every generation.
How is this machine built? There are three crucial structures--- the movable dactyl that rotates around a ball-shaped pivot joint at the base of the dactyl; a narrow flat blade inside the claw (called the opener apodeme) that attaches above the pivot; and a wide flat closing apodeme below the pivot. There are two layers of muscle that run from the inside of the claw’s shell to each of these thin apodemes, and these remain visible when you have removed the muscle. The muscle fibers tilt backwards so that upon contraction, they pull the apodemes backwards (to the right in the image below).
These two apodemes are internal extensions of the exoskeleton for the attachment of muscles. Because the apodemes attach on opposite sides of the pivot (fulcrum) of the joint, they pull the levers that operate the dactyl in opposite directions. When the muscles attached to the smaller (upper) apodeme contract, they open the claw, while contraction of the muscles attached to the larger (lower) apodeme close the claw.
The distance between the fulcrum and the attachment of the larger apodeme is much longer than it is for the smaller one (compare A with B, below), like an unequal see-saw. Any force exerted by pulling on the closing (as in “to close”) apodeme would be magnified greatly by the length of the lever arm A relative to that of the opener B. This is what every middle schooler learned (or should have) as mechanical advantage. Greater force comes at the expense of greater movement. Because lower apodeme pulls the dactyl closed, the closing force is multiplied several fold by the levers alone.
But in addition to this mechanical advantage, several times as much muscle is attached to the wide, closing apodeme, compared to the opener, multiplying the closing force several times more. I won’t bore you with the calculations, but combining levers and muscle areas, the closer muscles can exert 20 times as much force as the opener muscles. This is in line with the function of the claw because the crab is gripping, crushing, biting or cutting, and that takes a lot of force, whereas opening the claw encounters little resistance and doesn’t take much force.
The animation below shows these actions in motion. Watch the movement of the two apodemes as the claw opens and closes. The large apodeme is pulled to the right as the muscles contract during closure, while the small one moves to the left. The opposite is true during opening. You can also see how much farther the attachment of the large apodeme moves relative to the small one, revealing its mechanical advantage. The cropped animation below allows a closer view of these motions.
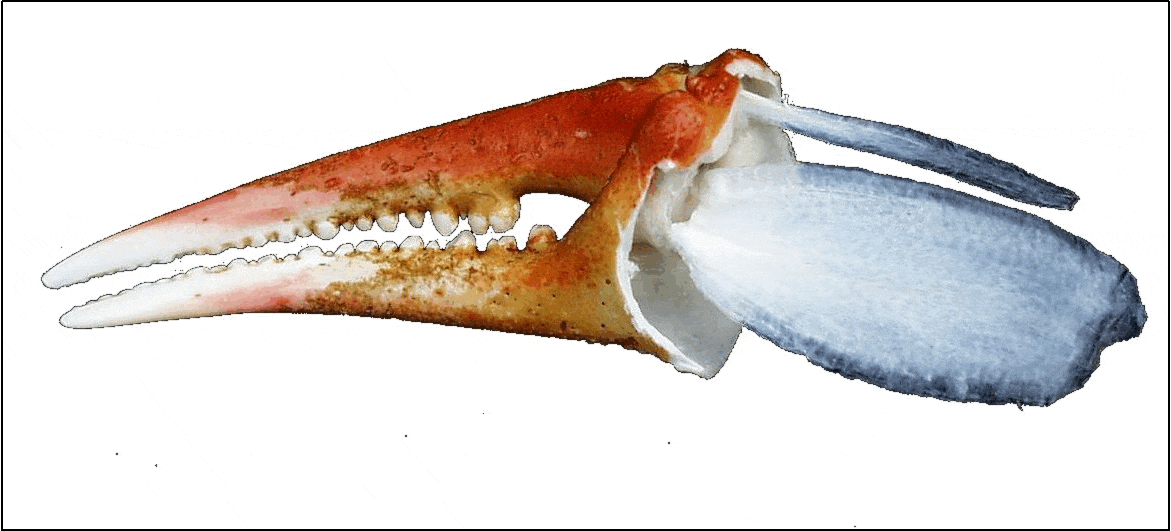
The same force/movement trade-off also occurs along the length of the dactyl. The greater the distance from the pivot (1), the less will be the force of closure, but the greater will be the range of motion, as in the picture below. For this reason, the crusher teeth (2) are located close to the pivot joint (1), an optimal compromise between force and range of motion. The dactyl tip (3) has the large range of motion needed for picking, nipping, scraping, and wiggling.
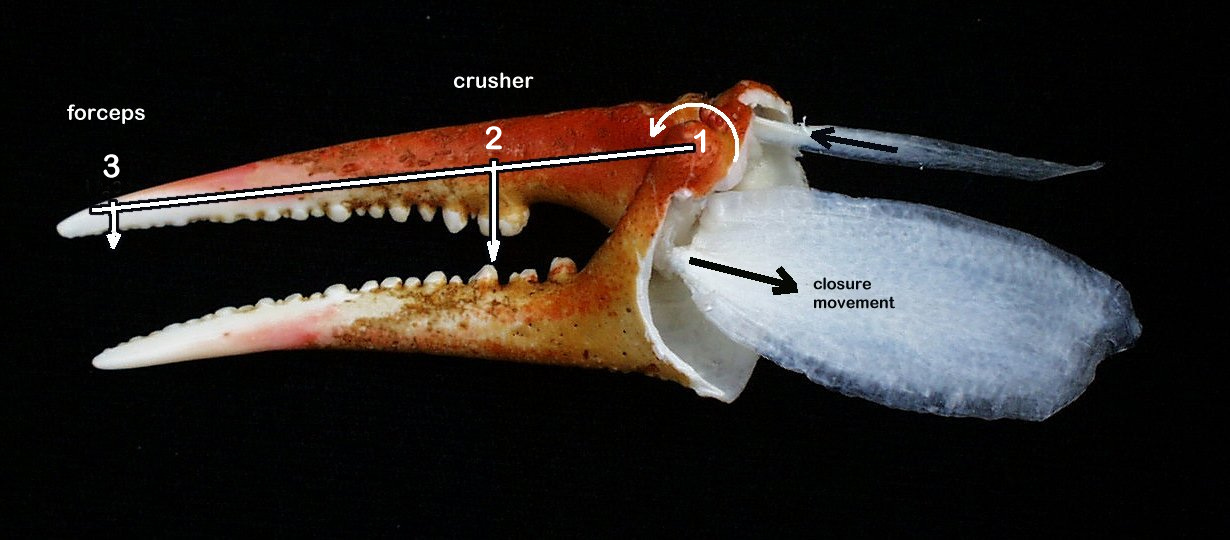
We are all machines. Like the crab claw, our bones are levers of various lengths and ratios, our joints are fulcra, and our muscles are pullers, turning contraction force into motion. Next time your party host serves blue crab claws, think about how the jaw that you just used operates on the same principle. Two very large, strong muscles originate on each side of your head and attach on your lower mandible. These muscles allow you to crack walnuts (or crab claws) if you so choose, and the molars you would use for that are closer to the pivot than the cutting teeth in the front. By comparison, the muscles that open your jaw are puny, running under your chin to the hyoid cartilage. No way would they allow you to crack walnuts. So, consider that the machine you are using is engineered like the machine you are eating--- each can be described by the location of the fulcrum, the relative length of the levers, the relative size of the muscles, the motion of the attachment points, and the mechanical advantage generated. Once you have understood that, and appreciate that this little machine is precisely built during the life of every crab, you will deserve another crab claw.
Hello Walter,
I think I'm good. I found some small samples of crab skeletons at our local beach (Chesapeake Blue Crab) and was able to play with those.
I understand how the hinges work now and should be able to put that together in 3D sculpting.
Thanks again for your awesome detail on that.
I'll let you know what I have once I get it to work.
:-)
Thank you! I'm working on animating/rigging this fascinating hinge in Blender on a 3D mesh that I got from a CT scan off the Smithsonian. I'm aiming to eventually 3D print this as a working piece that students can touch, look at and understand in real life. Thank you again for explaining this, no I finally understand how this works.