To start with a full disclosure, the Found Objects for this essay were originally found by a tiny ant, not by me. I found them secondarily, all piled up in the ants’ nests. But who is to say that Finding Objects is something only a person can do? Here we have an ant that does so, raising the question of why? I am not even sure why I collect objects, so figuring out why the ants do presents a challenging question, one that I set out to answer several years ago.
The ant in question is Pheidole adrianoi and is common in open, sandy sites in the coastal plains pine forests of Florida. I began working on this ant because it was so tiny, in fact, it is one of the tiniest ants in our fauna. Life is experienced radically differently at this tiny scale compared to our scale. Whereas our world is dominated by gravity and inertial forces such as momentum, that of tiny creatures is dominated by molecular forces such as viscosity, cohesion, adhesion, diffusion, and electrostatic charge. I wanted to imagine how I would experience the world if I were as tiny as this ant.
This ant is so tiny that if you really want to see it, you must get on your hands and knees and look for what appear to be tiny, moving apostrophes, and then follow these apostrophes back to where they disappear into the ground between grains of sand. You have then located a nest. If you keep watching, you might see a few workers come out of the entrance, walk a few millimeters and drop a grain or two of sand. They are busy excavating or just doing housework.
In our everyday lives, we experience a range of granularity in materials from smooth like sour cream to granular like sand and gravel. Because the ant is so small, the relative granularity of the sand in which it nests is extreme. The average grain of sand weighs more than the average worker. In effect, these ants nest in a pile of boulders. If you wanted to experience similar relative granularity, you would have climb a scree slope of huge boulders in the Sierra Nevada, slipping through the spaces between the boulders.
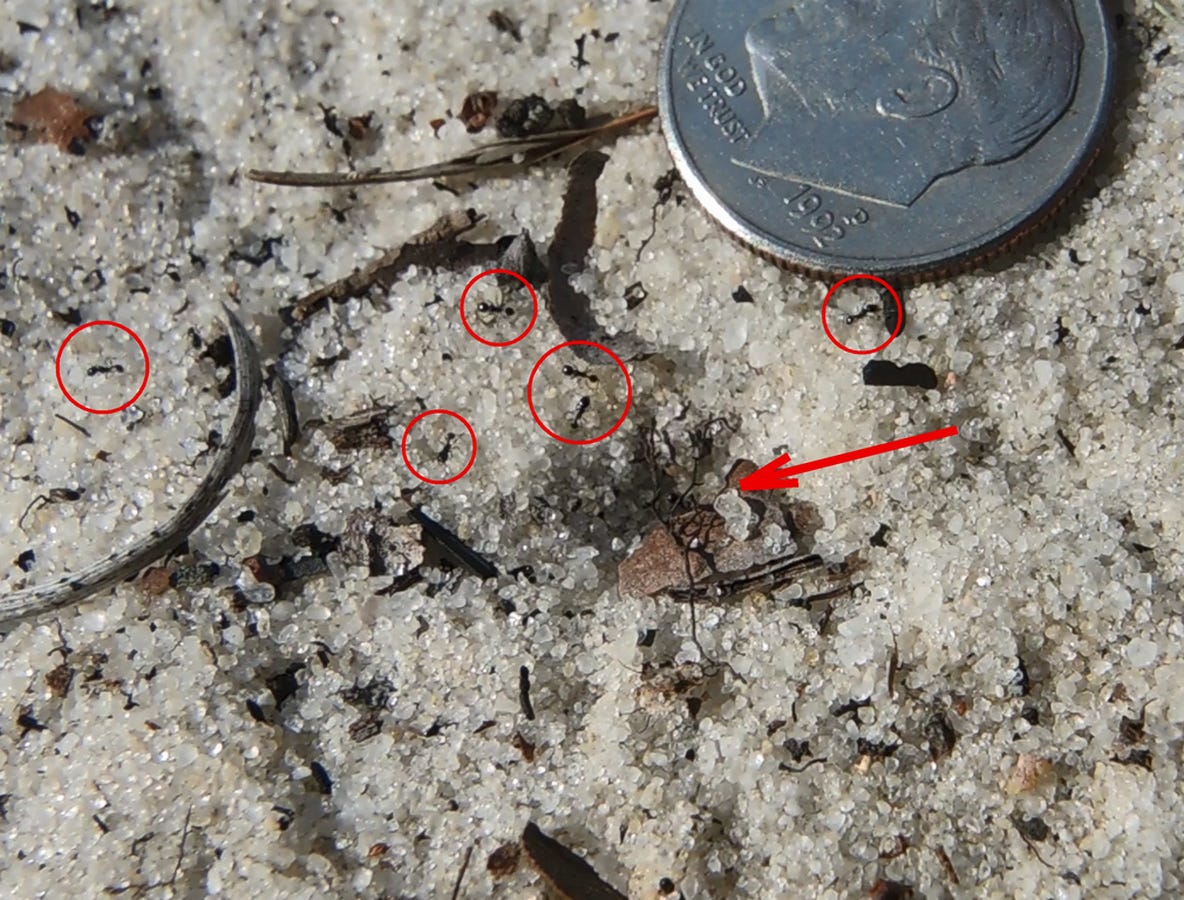
Because the ant is so tiny, it would seem like a losing proposition to consider digging up a nest, but, it isn’t very hard at all. With an aspirator or a hand vacuum, you remove the loose, dry sand on the surface, exposing a grey sand layer bound by fungus and rootlets. You will then spot a tiny hole from which an occasional worker will appear. This hole opens into a shaft that is just large enough to receive a longleaf pine needle. Push it down and it will come to a stop that tells you where the first chamber is (usually 2-6 cm). Now all you must do is remove the sand around the pine needle to expose the first small chamber at whose bottom will be another hole that is the continuation of the (always) vertical shaft. This time, the pine needle stops at 10 to 15 cm, and removal of the sand will expose the second, larger chamber. The floors and walls of this chamber will be partly black, contrasting with the white sand around it.
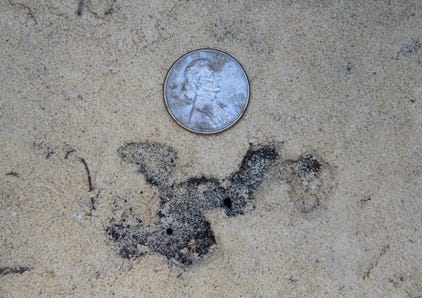
Again, cleaning the loose stuff with the hand vac reveals the continuation of the shaft, and you repeat the pine needle trick. This time it stops at 20 to 25 cm. Exposing the chamber reveals a complex entity with lobes, levels and side passages, all completely lined in black. Some of the side hallways are completely stuffed with trash (but that is a subject for another essay). You repeat the process to 40 cm to another larger, completely black chamber, and most of the ants are in it. The shaft does not continue, so this must be the bottom of the nest. It too is multi-tiered with dead end side chambers stuffed with trash.
But now finally, we come to the Found Objects that are the subject of this essay. These egg-shaped, black objects are stacked by the hundreds in windrows in some parts of the chamber. To repeat my disclosure, these were originally the ants’ Found Objects, and upon my excavation they became my Found Objects.
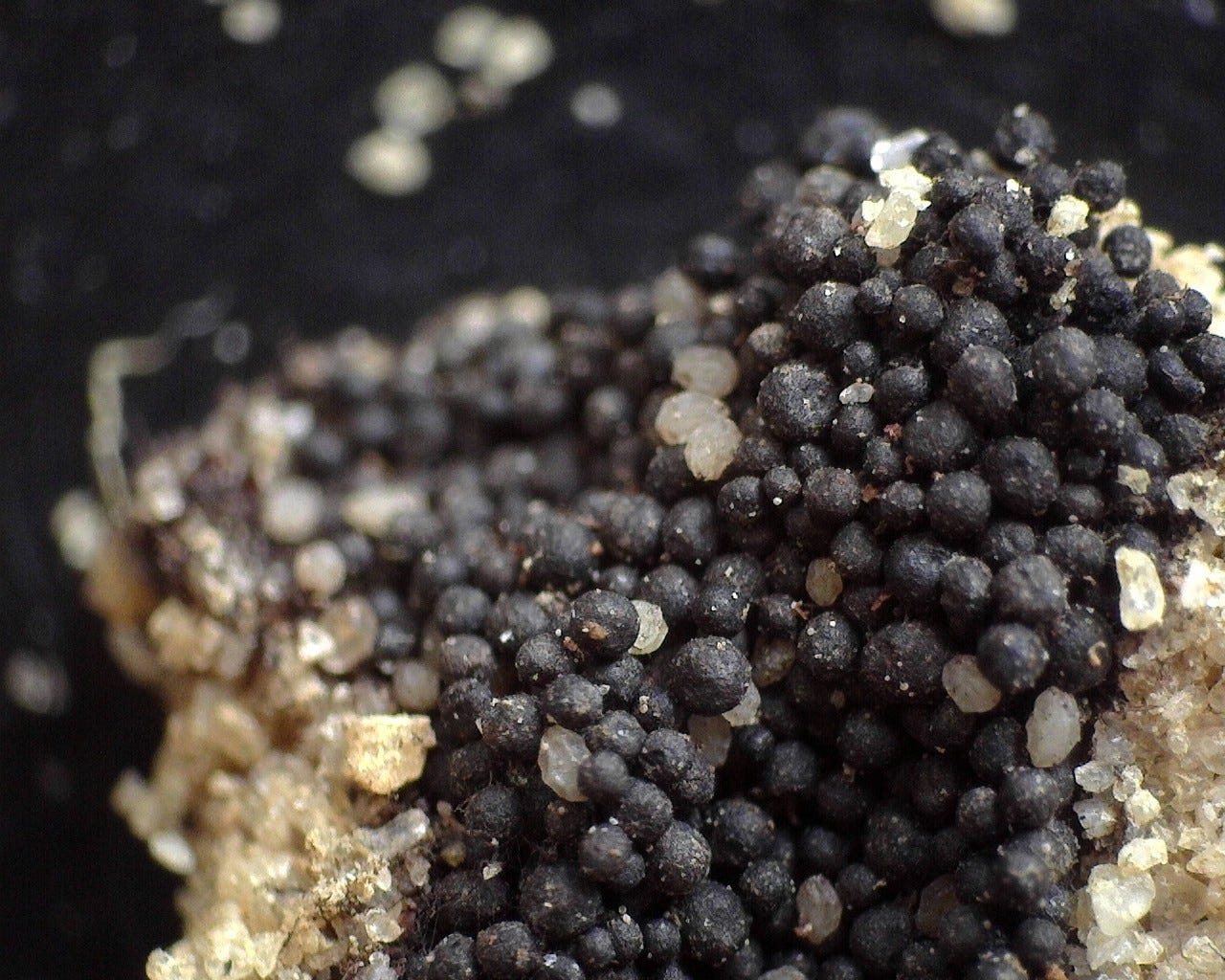
But what are these tiny things that measure 300 to 600 microns (0.3 to 0.6 mm)? An earlier note mentioned them to be seeds, but look as I might, they lacked seed traits, for example, a distinct seed coat and a hilum at which they were attached to the ovary. I inspected both whole and broken objects under a dissecting microscope, but this didn’t provide enough magnification to resolve the internal structure. Access to a scanning electron microscope (SEM) revealed that the objects had bumpy skin and a foam-like interior. In some, the foam cells seemed to be partly filled with a thick liquid.
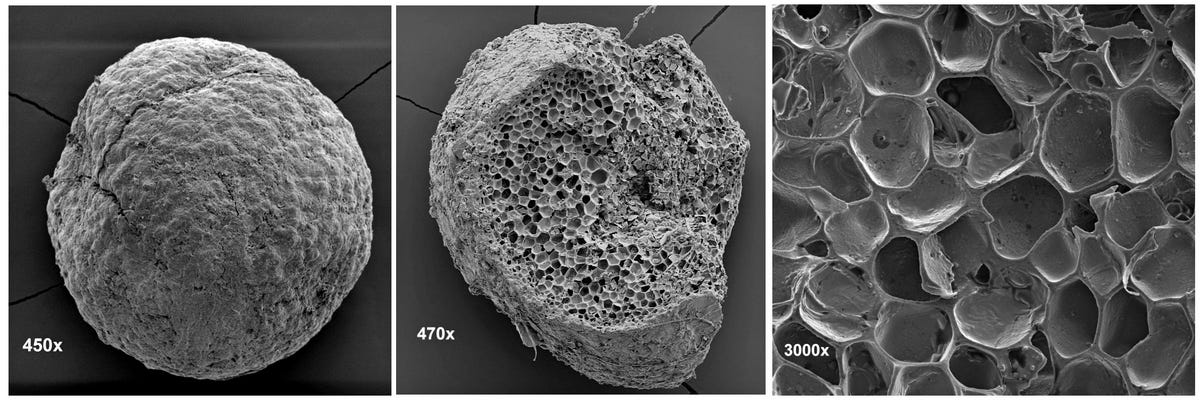
For several weeks I checked out various possibilities until I came upon an image on a website that looked exactly like my (our, if you include the ants) Found Objects. They were identified as “sclerotia”, the durable resting stages of a fungus that was symbiotic with the roots of plants, a so-called mycorrhizal fungus. Well, sure! And because I was working in a forest packed with pines, the Found Objects must be the sclerotia of pine mycorrhizal fungi! It didn’t take long to discover that the fungus Cenococcum geophilum was almost universally associated with pines all around the world, and that it was clearly not a single species, whatever that means in a fungus.
Eventually, I learned of a fluorescent dye that specifically stained fungal tissue, so I applied it to both whole and broken sclerotia. Under ultraviolet light, the interior of sclerotia glowed vivid blue, confirming their fungal nature.
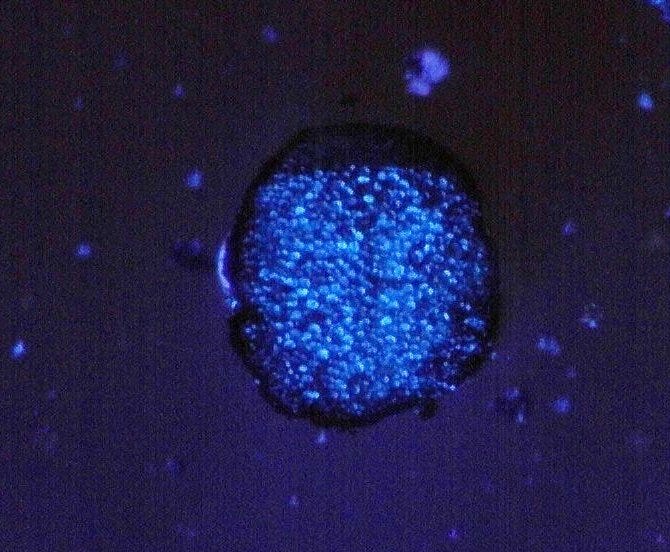
The origin of these sclerotia thus lay with the pine rootlets. In the sandy, nutrient-poor, highly weathered soils of the sandhills, the top 2 or 3 cm are loose sand, but beneath this thin shifting layer is a 5 to 10 cm thick layer of darker sand, tightly bound by a dense mat of pine rootlets and their mycorrhiza. A total of 85% of soil mineral nutrients are found in this layer, with deeper layers essentially sterile. This is the grim reality for pines struggling to make a living in this habitat— if they don’t manage to capture the nutrients that leach downward from the surface by a depth of 10 or 15 cm, they have lost them. The symbiosis of the pines and mycorrhiza in this thin layer makes it possible for both partners to thrive in this very sterile, challenging habitat in which most of the nutrients are in the biomass, not the soil.
As you might expect, the abundance of sclerotia in this dense zone of mycorrhiza-and-rootlets is several times as high as in higher or lower layers (10 to 20 sclerotia per gram of soil vs. fewer than 2 or 3). Oddly, the source of sclerotia for the ants is unlikely to be by direct excavation in this zone, as chambers in this rootlet layer are always very small. Various forces such a rain-splash, wind and animal tracks liberate a modest density of sclerotia into the loose surface layer, and this is likely where ants collect them.
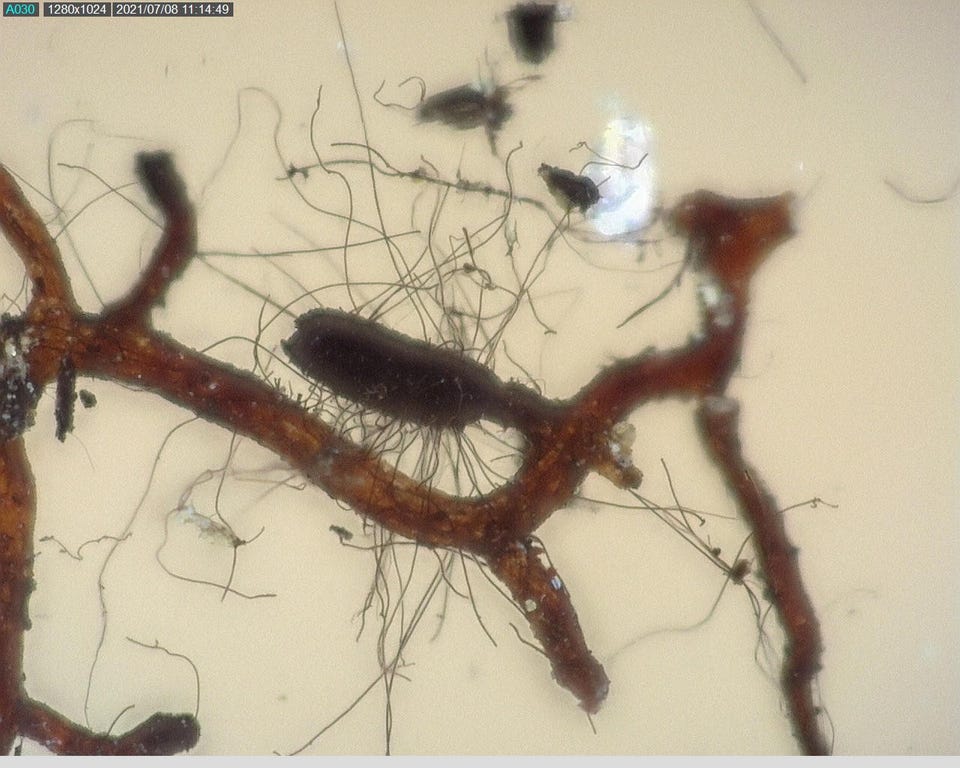
OK, so now we know about the pines and their fungal partner. I set up some glass-top colonies on my desk and could see that the ants collected sclerotia freely, and even broke some of them up, packing the pieces to the sides of the chambers. What’s in it for the ant that they should collect these sclerotia by the thousands? What do they do with them, and how does it benefit them? This is a much harder question to answer, one that generates stacks of hypotheses.
A first thought would naturally be that the ants eat them, but even when I offered broken, dyed sclerotia, there was no evidence that they did so directly. Maybe they farm them to eat the mycelia that grow from them? I surface-sterilized sclerotia and placed them on complete nutrient agar in Petri dishes. Abundant mycelia sprouted from some (but not all) sclerotia, and they took up eosin dye, but no eosin dye appeared in the ants—- there was no evidence that the ants ate these mycelia.
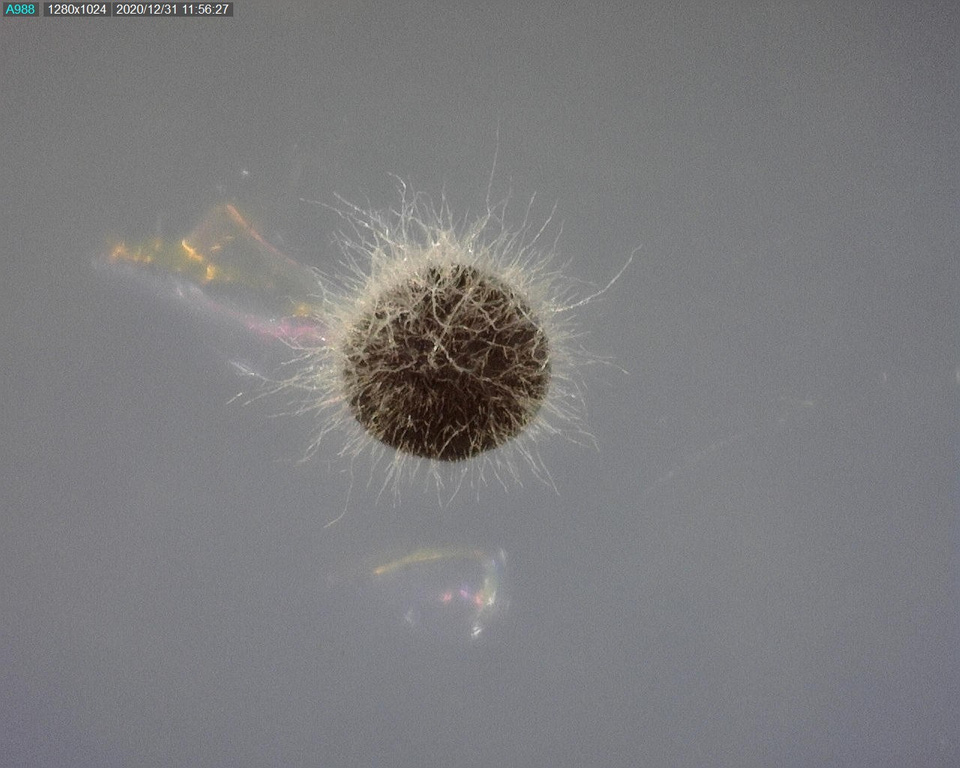
How about some “higher level” purpose? Something chemical, perhaps, such as inhibiting deleterious soil microorganisms? I inoculated agar plates with rinses of the soil in which the ants live and placed both whole and broken sclerotia in the carpet of bacteria or fungi. I could see no zones of bacterial growth inhibition, although there seemed to be mild inhibition of other fungi. Whether this was enough to be meaningful, I was unable to say.
The chamber linings were composed mostly of sclerotia fragments, with whole sclerotia often in piles that looked like supply depots (see image above). When I offered my pet desk colony sclerotia that had been marked with fluorescent printers’ ink, fluorescent fragments soon lined the chamber margins. Did the ants break up the sclerotia simply to produce a finer paving material, like crushing cobblestones into gravel, or might there be another reason?
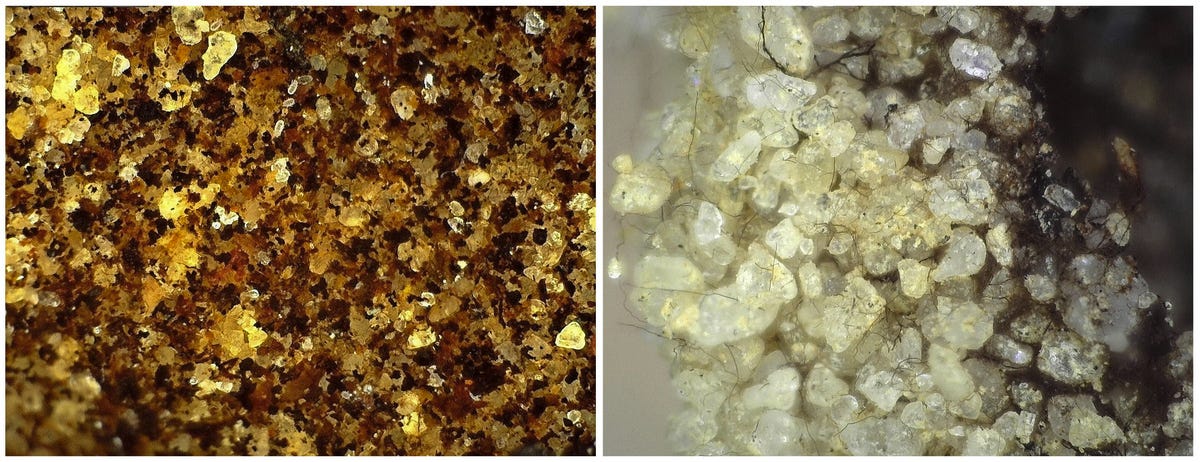
I set up some whole and broken sclerotia in damp sand between plates of glass and after a few days, stained the results with calcofluor dye to detect fungal mycelia. Under ultraviolet light, whole sclerotia remained dark, but the crushed pieces were swathed in a wispy, glowing blue fabric. Crushing has caused them to sprout copious mycelia. Another piece of evidence fell into place. The ants were breaking the sclerotia up in order to make them sprout, weaving the fragments and the surrounding sand together like a carpet.
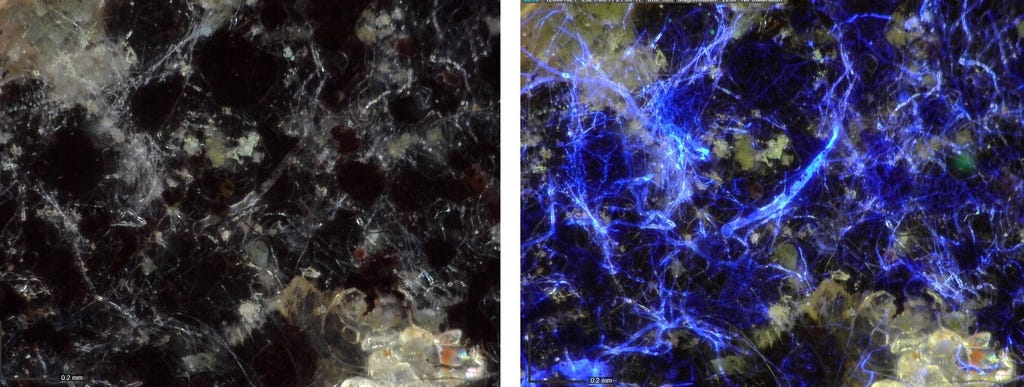
As a result of this binding together, the black chambers can be recovered whole, retaining their integrity despite considerable, though careful, handling. Teasing away peripheral sand produced chambers that remained hollow and intact. Viewed from a broken edge, mycelia penetrated from the lining of black fragments into the bright surrounding sand, tying multiple grain-widths of sand to the chamber linings.
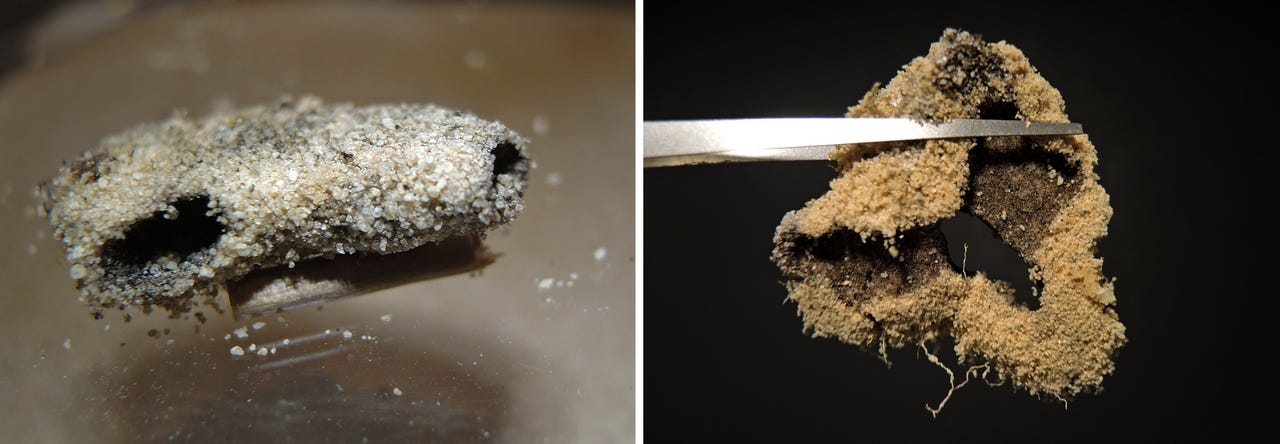
While science is good at answering What and How questions, it often struggles to answer Why questions. At this point, we know what the ants do and how they do it, but the ultimate Why question still eludes us. By this I mean what selective pressures lead to the evolution of this ant behavior? Did it prevent frequent chamber collapse in these droughty sands? Maybe, but in moist sand, chambers seem quite stable, and at the depth of the chambers, the sand is almost always moist. Perhaps cave-ins are indeed a challenge in the extreme granularity, the scree pile, in which the ants’ live. Are the black floors barriers against deleterious microbes? Maybe, but my inhibition tests were ambiguous, showing possible action against other fungi, but suggesting more sophisticated tests are needed. Do the sclerotia or their mycelia serve as food? Pretty unlikely. And of course there can be reasons that I have not yet thought of (and possibly never will).
As incomplete as is my current understanding of Why, the effect of this ant behavior is clear— it provides their chambers with remarkable stability and integrity, as well as a physical barrier in the form of carpeted floors, ceilings and walls. Several years of my observations hint at a possible, more subtle function. Each colony occupies several nests at once, and moves brood and workers among them, doing so for long periods of time. Perhaps carpeting the chamber walls with sclerotia fragments somehow gives nests a longer life, either through physical stability or by preventing invasion by other fungi. No doubt, as for most things in nature, there are probably multiple answers to the Why question.
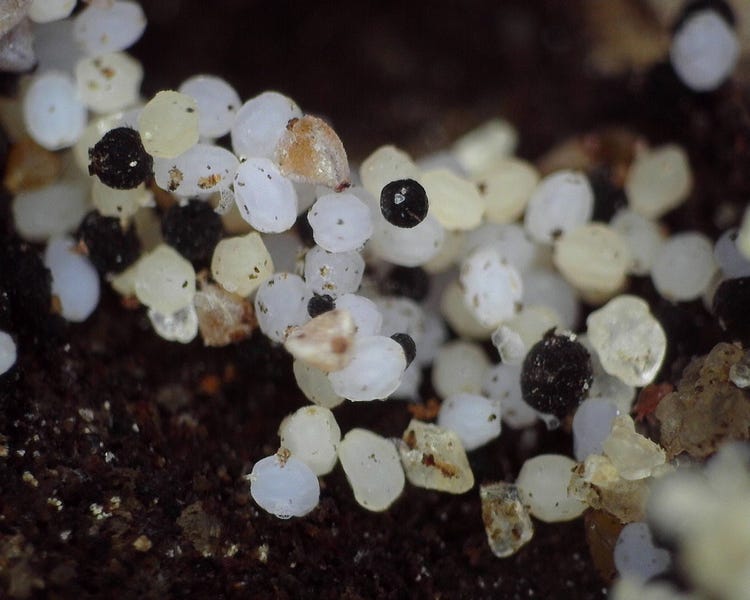
Finally, a postscript. In another little twist of doing science, several of the nests I excavated recently contained white objects the size of sclerotia, clearly organic rather than mineral (image above). What are these objects and why do the ants collect them? To answer this question, I would have to start this project all over again. So I ask, What now?
Stay tuned….
Your curiosity is matched by your patience and scientific discovery. Reminds me that even if I don’t understand exactly all the steps that life requires it is worth maintaining one’s curiosity and one’s skills.